Leafhoppers: Evolution and Biochemistry of Natural Nanoparticles
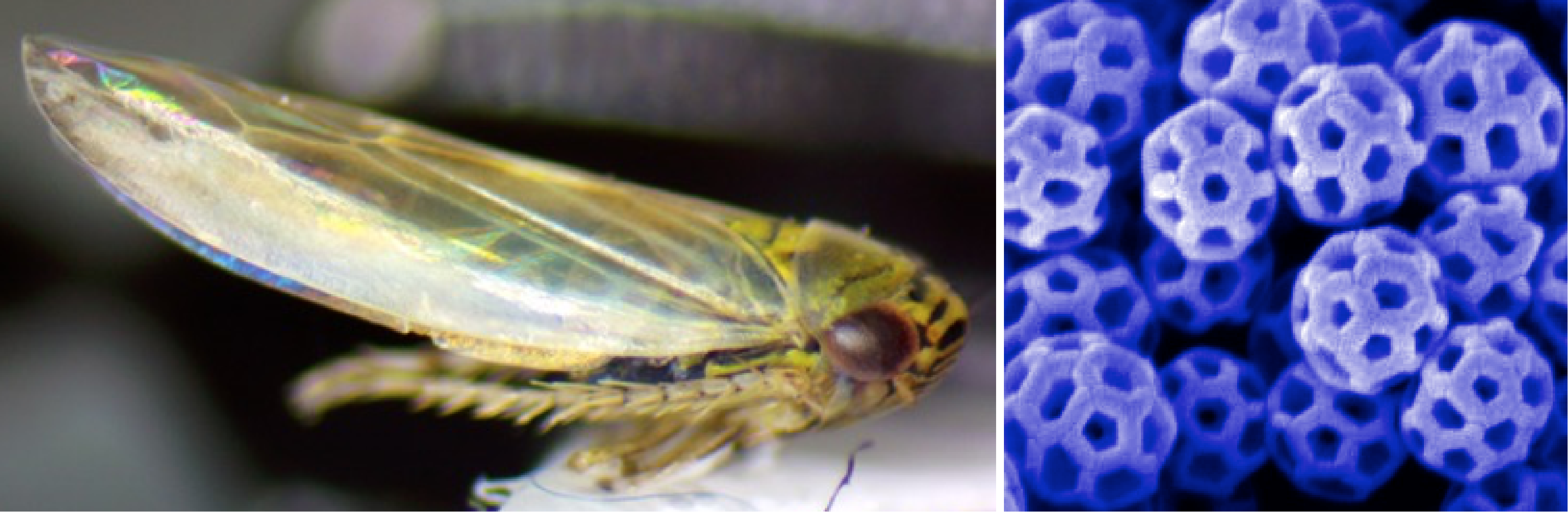
Leafhopper and SEM image of brochosomes on its wing
Leafhoppers produce unique nanostructures, called brochosomes, that they anoint onto their wings and sometimes eggs. Brochosomes have interesting materials properties, including superhydrophobicity and omnidirectional antireflectivity. With collaborating labs, we are characterizing natural variation in brochosome structures and performing comparative genomics to understand the molecular components that make up these structures, how they evolved, and their biological functions. We are also working towards genetically engineering symbionts of leafhoppers to create new tools for studying brochosomes and potentially enhancing their properties for various applications.
Representative Publications
Funding: ARO MURI, Stengl-Wyer Endowment
News: Tiny Insects Provide Inspiration for New Biomaterials
Identifying Mutations that Promote Bacterial Evolvability
Epistasis Poker. Early draws of cards that are more beneficial to the value of a poker hand (analogous to the most beneficial mutations in the eventual loser E. coli), prevent them from reaching the best hand after more cards are drawn.
Evolutionary potential (or
evolvability) is the capacity of an organism to generate descendants with increased fitness or new adaptive characteristics. Evolvability is a complex trait. It depends not only on how developmental and regulatory processes render underlying genetic changes into phenotypic variation, but also on the dynamics of how mutations arise and compete within a population. Mutator strains of microorganisms, which have elevated genomic mutation rates due to defects in DNA proofreading or repair pathways, can sometimes take over pathogen populations because they promote evolvability. However, little is currently known about how mutations affecting other cellular processes impact microbial evolvability or about how evolvability varies at each step in a typical adaptive trajectory.
We are reconstructing the dynamics of mutations in populations from the Lenksi 30-year
long-term evolution experiment (LTEE) with
E. coli to understand two cases of evolvability differences not related to mutation rates:
Eventual Winners and Losers. Bacteria that had evolved higher fitness early in one LTEE population reproducibly lost later to competitors with different, less-beneficial mutations when evolution was replayed many times from each starting point. Thus, the eventual losers apparently had favorable mutations that restricted further evolvability. We have found that loss of function mutations in the gene (
fabR) seem to be the major defining characteristic of the eventual losers and are investigating how interactions between this mutation and further adaptive steps differ from the alternative evolutionary pathways that won out in the LTEE.
Evolutionary pathway to Cit+ A metabolic innovation enabling aerobic citrate utilization only occurred in one of twelve populations and only after 30,000 generations of evolution. This new Cit+ trait was highly beneficial because it gave access to an untapped nutrient that was present for the entire duration of the LTEE at a much higher level than the glucose that these cells normally use as a sole carbon source. The lineage that evolved Cit+ appears to have evolved a potentiated genetic background that allowed it to access this innovation during the daily growth cycles of the LTEE. We have recently begun to characterize how these potentiating mutations remodeled central metabolism in ways that made achieving the very weak initial Cit+ phenotype beneficial enough to persist in the evolving population until it could be refined to enable full citrate utilization.
The long-term goal of this research is to further understand the mechanisms by which mutations can promote and reduce evolvability in order to be able to rationally make these changes to microbial genomes.
Representative Publications
Funding: NIH K99/R00
Experimental Evolution with Expanded Genetic Codes
Another way to potentially increase evolvability is to augment the chemical diversity of the building blocks available to an organism. We are evolving microorganisms, including bacteriophage, in the context of expanded genetic codes that re-code the amber stop codon into various non-canonical amino acids. This change increases the sequence-structure-function space available for evolution to explore. We are studying how evolution must compensate for the global change in how the information in an organism's genome is decoded, in cases where it is possible for an organism to survive this transition, and also how dependence on the new amino acid develops over time, such that an organism can no longer survive in the context of a normal genetic code. Finally, we are systematically examining how the unique chemistries of different amino acid building blocks are tolerated and uniquely useful for adaptation and using this technology to recapitulate the steps in a hypothesized pathway in which new post-translational modifications evolve in a series of adaptive steps from nonenzymatic metabolite damage to the proteome.
Representative Publication
Funding: Welch Foundation, NSF BEACON Center
AG3C: Associating Growth Conditions with Cellular Composition
We are part of a collaboration of eight labs (including experimental biologists, computational biologists, and mathematicians) that is collecting and analyzing comprehensive transcriptomics, proteomics, lipidomics, and metabolic flux data from bacterial samples grown under different conditions. The aim is to solve the "inverse problem" of predicting the conditions that were used to culture a microbial sample from measurements in these high-dimensional data sets (
AG3C Website). Our lab is primarily responsible for creating the microbial samples and using RNA-seq to examine RNA expression levels.
Representative Publication
Funding: DoD Army Research Office
Discovering Functional Nucleic Acid Families by Deep Sequencing and Fold Sampling
A final way that we are studying how to improve the potential of evolutionary methods is in systems for the in vitro selection of nucleic acid molecules. Nucleic acids with random sequences often misfold into inactive structures that are kinetically trapped. That is, they become stuck and cannot unfold to rearrange into other, potentially active, structures on a reasonable timescale. It is possible that this potential for misfolding, which is known to occur for even natural ribozymes, limits the recovery of new families of functional nucleic acids (aptamers, ribozymes, deoxyribozymes, riboswitches) by laboratory evolution methods that select for functional molecules from pools of 10
13–10
15 molecules with randomized sequences. We expect this problem to more greatly affect longer nucleic acid sequences, which have the capacity for folding into elaborate three-dimensional structures that achieve more rapid rates of catalysis or tighter binding affinities. We are currently using next-generation deep-sequencing techniques and methods to allow a single nucleic acid strand to have multiple chances to fold correctly to pass selection to see if they can enhance our ability to recover more complex families of functional nucleic acids.
Representative Publications
Funding: Welch Foundation
Evolution Experiments with Digital Organisms
Populations of self-replicating computer programs mutate and compete for CPU cycles in the Avida artificial life system. This environment for digital organisms has been used to ask fundamental questions that transcend the substrate that is evolving. The speed of digital evolution experiments, transparency of the underlying mechanics, and ability to manipulate and record every aspect of the evolutionary process make it possible to more readily explore cause and effect relationships in Avida than in natural systems. I have extensive experience with Avida. My lab will use Avida to complement studies in biological systems: both as a way of more clearly illustrating and exhaustively characterizing phenomena of interest and as a source of new candidate laws and insights to motivate new lines of experimental inquiry.
Resources